A simple rule drives the evolution of useless complexity
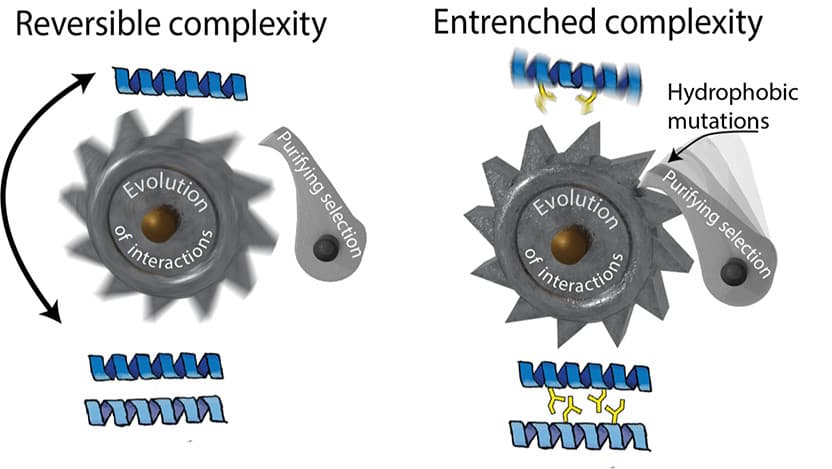
A new study at the University of Chicago has shown that elaborate protein structures accumulate over deep time even when they serve no purpose, because a universal biochemical property and the genetic code force natural selection to preserve them. The work was published on Dec. 9, 2020 in Nature.
Most proteins in our cells form specific complexes with other proteins, a process called multimerization. Like other kinds of complexity in biology, multimers are usually thought to persist over evolutionary time because they confer some functional benefit that is favored by natural selection.
“How complexity evolves is one of the great questions of evolutionary biology,” said senior author Joseph Thornton, PhD, professor of human genetics and ecology and evolution at the University of Chicago. “The classic explanation is that elaborate structures must exist because they confer some functional benefit on the organism, so natural selection drives ever-increasing states of complexity. Clearly in some cases complexity is adaptive, like the evolution of the eye: complex eyes see better than simple ones. But at the molecular level, we found that there are other simple mechanisms that drive the build-up of complexity.”
The research team, led by Thornton and University of Chicago postdoctoral fellow Georg Hochberg, PhD, set out to study the evolution of multimerization in a family of proteins called steroid hormone receptors, which assemble into pairs (called dimers).
They used a technique called ancestral protein reconstruction, a kind of molecular “time travel,” Thornton said, that allowed them to recreate ancient proteins in the lab and experimentally examine how they were affected by mutations that happened hundreds of millions of years ago.
To their surprise, they found that the ancient proteins functioned no differently when assembled into a dimer than if they had never evolved to dimerize at all. There was nothing useful or beneficial about forming the complex.
The explanation for why the dimeric form of the receptor has persisted for 450 million years turned out to be surprisingly simple. “These proteins gradually became addicted to their interaction, even though there is nothing useful about it,” explained Hochberg, who is now a group leader at the Max Planck Institute in Marburg, Germany. “The parts of the protein that form the interface where the partners bind each other accumulated mutations that were tolerable after the dimer evolved, but would have been deleterious in the solo state. This made the protein totally dependent on the dimeric form, and it could no longer go back. Useless complexity became entrenched, essentially forever.”
The researchers showed that simple biochemical, genetic and evolutionary principles make entrenchment of molecular complexes inevitable. The genes that code for every protein are subject to a constant hail of mutations over generations, many of which would disrupt the protein’s ability to fold up and function properly. A form of natural selection called purifying selection removes these deleterious mutations from the population.
Once a protein evolves to multimerize, the parts that form the interface can accumulate mutations that would be deleterious if the protein were in the solo state, so long as they can be tolerated in the multimer. Purifying selection then entrenches the complex form, preventing a return to the solo state.
The researchers showed that a simple and universal rule of biochemistry underlies entrenchment. Proteins are made up of amino acids, which may be water soluble, or hydrophobic, meaning they dissolve easily in oil but not water. Usually, proteins fold so the water-soluble amino acids are on the outside and the hydrophobic amino acids are on the inside. Mutations that make a protein’s surface more oil soluble impair its folding, so purifying selection removes them if they occur in solo proteins.
If the protein evolves to multimerize, however, those hydrophobic amino acids on the interface surface are hidden from water, and become invisible to purifying selection. The multimer is then entrenched, because returning to the solo state would expose the now-oil-soluble and deleterious interface.
This “hydrophobic ratchet” appears to be universal. The researchers analyzed a massive database of protein structures, including hundreds of dimers and related solo proteins, and found that the vast majority of interfaces have become so hydrophobic that the dimeric form is deeply entrenched.
This mechanism, operating on thousands of proteins over hundreds of millions of years, could drive the gradual accumulation of many useless complexes inside cells.
“Some complexes surely have important functions, but even those will be entrenched by the hydrophobic ratchet, making them harder to lose than they would otherwise be,” Hochberg said. “With the ratchet constantly operating in the background, our cells have probably built up a massive stock of entrenched complexes, many of which never performed a useful function, or long ago ceased to do so.”
Future directions include investigating whether or not interactions other than multimerization may be the result of entrenchment. “This was a story about proteins dimerizing with other copies of themselves, which is a super common process,” said Thornton. “But there are lots of other interactions in cells, and we think it’s possible that some of those may have accumulated during evolution because of a similar kind of acquired dependence on molecular complexity.”
The study, “A hydrophobic ratchet entrenches molecular complexes,” was supported by a Chicago Fellowship and the National Institutes of Health (R01GM131128 and R01GM121931). Additional authors include Brian P.H. Metzger of the University of Chicago, Yang Liu and Arthur Laganowsky of Texas A&M University, and Erik G. Marklund of Uppsala University.