Molecular matchmakers: Proteins help neurons select the correct partner

The nervous system of an animal can consist of up to billions of neurons that connect with each other to form circuits that shape behavior, coordinate movements, formulate thoughts and respond to the environment. But neurons can’t link up with just any partner. They have to find the appropriate matching partner to form a synapse and make a lasting connection.
These connections are directed by proteins on the surface of neurons and their respective partners. The proteins provide information to help neurons find each other, like matching the section and row numbers on a ticket stub to identify your seat in a stadium. Scientists still have a lot to learn about how these proteins work, but researchers at the University of Chicago have nailed down some proteins that help establish connections.
In back-to-back studies published in the journal eLife, Robert Carrillo, PhD, assistant professor in the Department of Molecular Genetics and Cell Biology, describes two groups of proteins that play a key role in motor neuron connectivity in fruit flies. One of the studies, spearheaded by Engin Özkan, PhD, assistant professor in Biochemistry and Molecular Biology, examines the “lock-and-key” structure of these proteins and how disrupting this interaction can alter connections. The second describes how they interact with each other to form circuits in the motor system.
Making connections and forming circuits
A neuron consists of a cell body with several short filaments branching out from it called dendrites. One longer fiber, called the axon, extends much farther and branches into several terminals that connect to another neuron or other targets to form a synapse, the connection that allows neurons to communicate with each other.
The axon terminal’s surface is labeled with different “presynaptic” proteins that help it find the corresponding “postsynaptic” label on its partner. Carrillo and staff scientist James Ashley, PhD, focused on two sets of binding proteins called Dprs and DIPs. Genetic analyses showed that the genes that produce these proteins were expressed in unique combinations in the motor neuron-muscle circuit of flies.
“We got a little lucky in this study because it was a simple example, even for fruit flies,” Carrillo said. “More likely, neurons will express combinations of these tags to serve as barcodes, not just one neuron expressing one tag and its target expressing the other.”
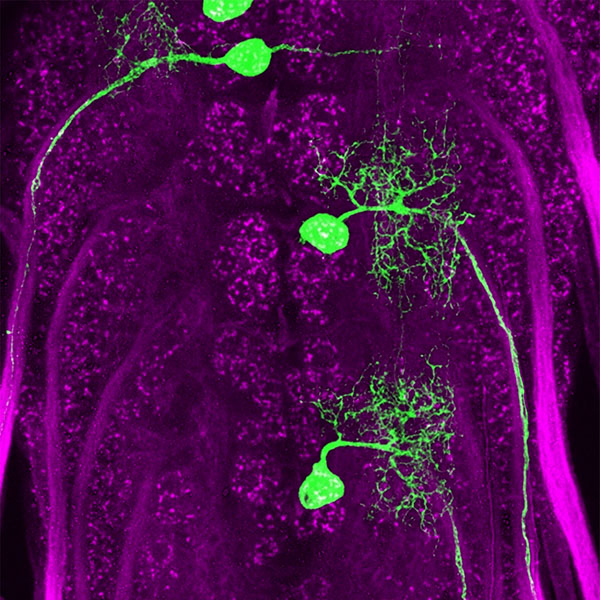
One pair of proteins from these groups, Dpr10 and DIP-α, turned out to be crucial for forming neuromuscular circuits during embryonic development. DIP-α is expressed by motor neurons and Dpr10 is expressed by postsynaptic targets in the muscle. These motor neurons normally make connections with multiple targets, but when the researchers knocked out the genes for either one of these proteins, the neural circuit was miswired. Some connections were never established, while other connections formed properly. This Dpr-DIP pair appears to be required for some connections but not others, suggesting the neurons have different barcodes for different targets.
“Normally knocking out a gene important for circuit formation causes miswiring in several circuits, so this is the first time we’ve identified a simple connection that relies on two interacting proteins,” Ashley said.
Carrillo said he wants to determine whether the mechanism identified in this simple neuromuscular circuit in flies is more broadly applicable as a basic mechanism for all neural connectivity. Many neurological diseases and disorders are caused by miswiring of the nervous system. If researchers better understand how that happens, they could develop treatments to intervene and stabilize those connections.
“When you think about it, these little connections form circuits that lead to every output, all our thoughts and every behavior,” Carrillo said. “It’s what makes us, us, and if things go wrong that little thing can become a big problem.”