Bacteria use different strategies to divide and survive under stress

Under laboratory conditions, many common bacteria reproduce and divide into symmetrical halves. In the real world with limited resources, however, conditions aren’t always ideal for this kind of carefully planned growth.
A new study by scientists from the University of Chicago shows how cyanobacteria (bacteria that produce energy through photosynthesis like plants) change the way they grow and divide in response to different levels of light. With typical light conditions, the cells remain relatively short and divide symmetrically. But as light dims, the cells grow longer and divide unevenly, resulting in two daughter cells of different lengths. The researchers believe this may be a survival strategy that evolved to help these bacteria survive in less than ideal conditions.
“In the real world, most bacteria are living in some growth limited, nutrient poor condition and they just get an occasional puff of something they can eat,” said Michael Rust, PhD, associate professor of molecular genetics, cell biology and physics, and senior author of the study. “Probably what evolution is selecting for is the ability to tolerate starvation for a long time and use those rare resources as efficiently as possible.”
In the new study, featured as the cover article in the latest issue of Cell Systems, Rust and Yi Liao, PhD, a postdoctoral researcher in his lab, used time-lapse imaging to track cell division in Synechococcus elongatus, a rod-shaped cyanobacterium. The researchers saw that under dim light conditions, which cause stress for cells by limiting their energy source, the S. elongatuscells grow longer than usual. When the lights are turned back up, cells shorter than eight micrometers still divide symmetrically, but above this length the divisions become uneven, typically producing one short daughter (about three micrometers) and a longer one.
In many bacteria, the position where a mother cell splits into two is controlled by something called the Min system, a group of proteins that moves around inside the cell. In a typical short cell, one of the proteins, MinC, pools in one end and then every few minutes moves to the opposite end. As they bounce back and forth, the MinC proteins spend more time at the ends and less at mid-cell, like squeezing a water balloon in the middle and wiggling it back and forth. Since MinC inhibits cell division, this oscillation creates a sort of weak point in the middle where the cell can divide.
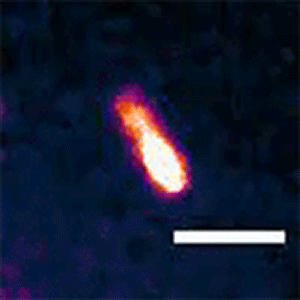
To see whether the Min system is also responsible for asymmetric divisions seen in longer cells, Liao and Rust tagged the MinC protein with a fluorescent marker that lets them follow its movements. They found that the Min system exhibits different patterns depending on the cell length. The proteins kept up a characteristic, back-and-forth oscillatory pattern in short cells, but they formed a variety of dynamic patterns in longer cells, including multi-band oscillations, traveling waves, and other more complex patterns. In all of these patterns in long cells, a region without MinC was always seen about three micrometers from the end of the cell, allowing cells to divide off-center and make a short daughter of a specific size.
“We think the patterns change because it's geometrically sensitive, so it can adapt to a changing cell size,” Liao said. “The ability to form these different patterns allows both shorter cells to divide symmetrically and longer cells to produce short daughter cells.”
Rust and Liao said they aren’t sure why bacteria divide into different lengths under stressful conditions or whether one length provides an advantage over another. It could be that smaller cells are able to maneuver better to find resources. Elongating themselves could give the cell more surface area to absorb light in dim conditions. There is even evidence that length is a defense mechanism for pathogens, because it’s harder for an immune cell to engulf a really long bacterial cell.
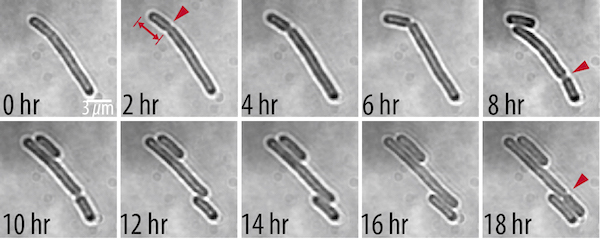
Either way, Rust said understanding how bacteria grow and divide under real world conditions could help strategies for using antibiotics. For example, cells might need to be a certain length to be able to infect someone, or they just elongate themselves and don’t divide as a way of riding out the tough times. Researchers could use this to their advantage and design ways to interfere with the Min system and disrupt the bacteria’s tricks for survival.
“There's this whole hidden world of the biology of suboptimal conditions,” Rust said. “We can understand how organisms are growing and thriving in difficult circumstances that may unveil new rules of how life behaves.”
The study, “The Min oscillator defines sites of asymmetric cell division in cyanobacteria during stress recovery,” was supported by the National Institutes of Health and the Howard Hughes Medical Institute.

Michael Rust, PhD
Michael Rust, PhD, is an associate professor in the Department of Molecular Genetics and Cell Biology and the Department of Physics. His research focuses on understanding how the behavior of living cells is produced by the biochemical interactions of many non-living molecules.
Learn more about Dr. Rust